P 9: M. Engstler / F. Groeber-Becker
Development of tsetse fly-transmitted African trypanosomes in human skin tissue models
State of the art
African trypanosomes are a major threat to health and the economy in large parts of sub-Saharan Africa. The unicellular parasites not only cause the deadly human sleeping sickness but also the widespread disease nagana in cattle. Trypanosomiasis is not a tourist’s plague but a prototypical neglected tropical disease of poverty. The few drugs available are difficult to administer and very toxic to the patient. Diagnosis of sleeping sickness is underdeveloped and painful. On the other hand, trypanosomes have attracted the attention of modern biology for many decades. Major discoveries in molecular cell biology and biochemistry have been made using trypanosomes as a model. Despite this, significant parts of the trypanosome life cycle and many aspects of the human infection remain enigmatic, including the invasion of the human brain and the parasite’s development within the transmitting tsetse fly.
Another example is the first site of infection, the trypanosomal chancre, a local skin inflammation caused by the tsetse’s bite. The blood-sucking fly injects the parasites into the host’s subcutaneous tissue, where they proliferate for several days, before they enter the draining lymphatic system and reach the human circulation. Very little is known about the interplay of factors from vector, host and parasite within the trypanosome chancre.
Previous Work
African trypanosomes feature a dense cell surface coat of variant surface glycoproteins (VSG) that protects the parasites against the host’s immune attack. Our research has focused on the synthesis, maintenance and function of the VSG coat [1]. We have shown that the trypanosome plasma membrane is recycled with unprecedented kinetics through the flagellar pocket, a small invagination of the cell surface [2]. The high endocytosis rate becomes effective during antibody clearance, a highly efficient process, by which the directionally swimming parasite exploits hydrodynamic forces to specifically drag antibody-bound VSGs into the flagellar pocket [3]. Antibody clearance requires directional motility and the presence of blood cells [4, 5]. The trypanosome genome contains hundreds of different VSG genes, of which only one is expressed at any given time. We have detailed a molecular mechanism that initiates antigenic variation, thereby establishing a surprising link between VSG switching and parasite development to the insect vector stages [6]. Recently we have started investigating the complex passage of trypanosomes through the tsetse fly. For this purpose, we established a tsetse fly colony in Würzburg.
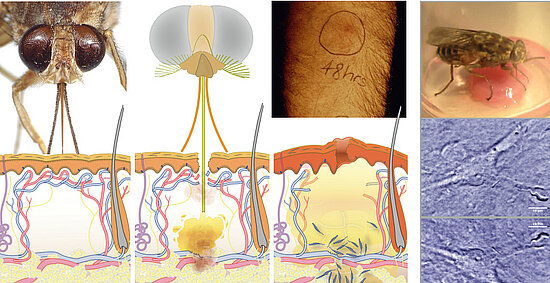
Figure: The tsetse fly injects trypanosomes into the human skin, where the parasites cause a local inflammation, the trypanosome chancre.
Work Plan
The natural vector transmission of African trypanosomes involves the deposition of the parasites into the host skin at the site of the fly’s bite [7]. The inoculum from a vector challenge is complex, containing multiple factors – from fly and parasite – that modulate the host environment at the site of the bite. Thus, vector transmission differs significantly from experimental syringe passage. The fly has to salivate to feed, no matter if infected or not. Hence, tsetse saliva could be considered as a parasitic niche, and trypanosome development might be dependent on saliva-derived signals. Experimental approaches to host skin as first site of infection have been obstructed by the lack of appropriate animal models. We suggest that artificial human skin could provide a versatile model system for early trypanosome infections.
As proof-of-principle we have shown that infected tsetse flies probe on engineered skin pads, thereby injecting infective metacyclic trypanosomes into the skin tissue, where the parasites persist and proliferate for several days. We now plan to establish vascularized skin models for tsetse infection together with Florian Groeber and Heike Walles. We will detail the parasite’s cell biology in the skin using advanced fluorescence imaging of transgenic parasites. Trypanosome development to the bloodstream stage will be observed as changes of VSG expression and FPGA-assisted long-term tracking will monitor the actual entry into the engineered vascular system.
Dual RNA-Seq should allow us to distinguish parasite and saliva-induced variations in gene regulation. Metabolite profiling will be used for detailing changes in saliva composition in the presence of a developing trypanosome population. Whole genome RNA interference and comparative proteomics will be performed at later stages of the project for systematic identification of potential genetic regulators of trypanosome skin infection. The optimized skin infection model will ultimately serve as a test platform for skin-compatible formulation of drug candidates against sleeping sickness. Here, the special focus is on the trypanosome quorum sensing factor SIF.
References
- Engstler et al. (2004) Kinetics of endocytosis and recycling of the GPI-anchored variant surface glycoprotein in Trypanosoma brucei. J Cell Sci, 117,1105-1115. PubMed
- Overath, P. and M .Engstler (2004) Endocytosis, membrane recycling and sorting of GPI-anchored proteins: Trypanosoma brucei as a model system. Mol Micro 53, 735-44. PubMed
- Engstler et al. (2007) Hydrodynamic flow-mediated protein sorting on the cell surface of trypanosomes. Cell 131, 505-515. PubMed
- Heddergott et al. (2012) Trypanosome motion represents an adaptation to the crowded environment of the vertebrate bloodstream. PLoS Pathog 8(11), e1003023. PubMed
- Uppaluri et al. (2012) Flow loading induces oscillatory trajectories in a bloodstream parasite. Biophysical Journal 103: 6, 1162-1169. PubMed
- Batram et al. (2014) Expression site attenuation mechanistically links antigenic variation and development in Trypanosoma brucei. eLife 2014;3:e02324. PubMed
- Engstler, M. and M. Boshart (2004) Cold shock and regulated surface protein trafficking convey sensitization to inducers of stage differentiation in Trypanosoma brucei. Genes & Development, 18, 2798-2811. PubMed